Why Do Some Buildings Not Collapse During an Earthquake?
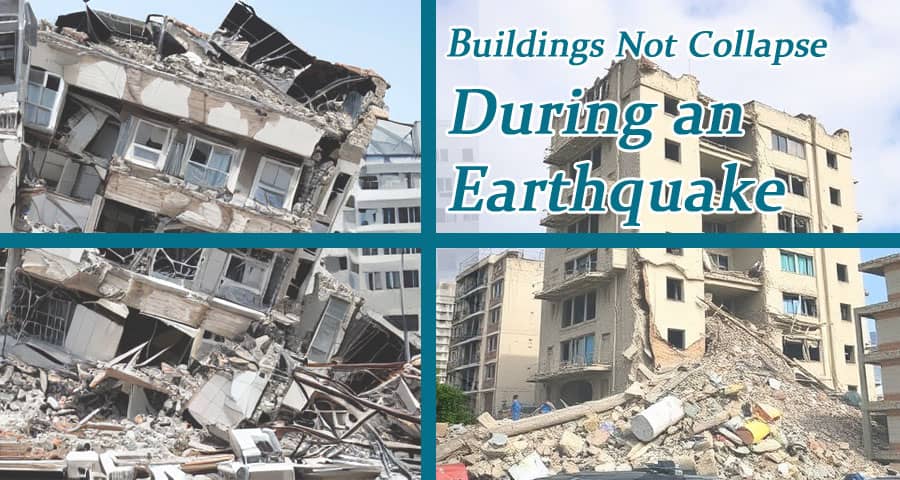
Earthquakes are natural disasters that test the limits of human engineering. While some structures crumble under seismic stress, others remain standing, often saving countless lives. The resilience of certain buildings lies in the interplay of design, materials, and technology.
The Science of Earthquake Resistance
Understanding Seismic Forces
During an earthquake, seismic waves cause the ground to shake horizontally and vertically. These movements generate forces that buildings must endure. Structures not designed for such stress can succumb to these forces, leading to catastrophic failure.
Engineers address this by designing buildings to withstand lateral forces, which are the primary cause of collapse. This involves calculating the potential seismic forces a structure may face and incorporating countermeasures to resist them.
Key Design Principles for Earthquake-Resistant Buildings
Flexible Structures vs. Rigid Structures
A major factor in earthquake resilience is a building's flexibility. Rigid structures are prone to cracking and collapsing when subjected to seismic forces. Flexible designs, on the other hand, allow buildings to sway with the motion of the ground, dissipating energy and reducing the likelihood of structural failure.
Materials like reinforced steel and specially treated concrete provide both strength and elasticity, enabling buildings to bend without breaking.
Base Isolation Technology
Base isolation is one of the most effective methods of protecting buildings during earthquakes. This involves placing isolators, such as rubber bearings or sliding plates, between the building's foundation and its structure. These isolators absorb and redirect seismic energy, allowing the building to remain relatively motionless while the ground moves beneath it.
This technology has been successfully implemented in hospitals, government buildings, and skyscrapers worldwide, reducing earthquake damage significantly.
Damping Systems
Damping systems are engineered to absorb and dissipate seismic energy. These systems act like shock absorbers in a car, reducing the amplitude of vibrations.
Common types of damping systems include:
- Tuned Mass Dampers (TMDs): Large, heavy pendulums installed at the top of buildings to counteract seismic motion.
- Viscous Dampers: Devices filled with fluid that absorb energy and reduce vibrations.
- Friction Dampers: Mechanisms that dissipate energy through controlled sliding friction.
By incorporating these systems, buildings can withstand even severe earthquakes without structural collapse.
Reinforced Structural Components
Reinforcing critical structural elements is crucial for earthquake resistance. Key components include:
- Beams and Columns: These must be designed to support lateral loads, with reinforcements like steel bars providing extra strength.
- Walls: Shear walls, made of reinforced concrete or steel, resist horizontal forces effectively.
- Foundations: Deep foundations anchored into bedrock provide stability and reduce the risk of overturning.
Proper reinforcement ensures that the building's skeleton remains intact, even under extreme stress.
The Role of Materials in Earthquake Resistance
High-Strength Concrete
Modern earthquake-resistant buildings often use high-strength concrete, which is less prone to cracking and offers better durability. Adding fibers, such as polypropylene or steel, further enhances its resilience.
Steel Reinforcement
Steel is a vital material in earthquake-resistant construction due to its flexibility and tensile strength. When combined with concrete, it creates a composite material known as reinforced concrete, which can endure both compressive and tensile forces.
Innovative Materials
Engineers are now exploring advanced materials like carbon fiber-reinforced polymers (CFRPs) and shape-memory alloys. These materials offer superior strength, flexibility, and self-healing properties, making them ideal for seismic applications.
Architectural Design Considerations
Symmetry and Regularity
Buildings with a symmetrical and regular layout are less likely to collapse during earthquakes. Asymmetric designs can lead to uneven distribution of forces, increasing the risk of failure.
Lightweight Construction
Using lightweight materials for non-structural components, such as walls and partitions, reduces the overall load on the building. This minimizes the forces exerted during seismic events.
Low Center of Gravity
A building's stability improves with a lower center of gravity. Engineers achieve this by concentrating heavier components, such as machinery and structural reinforcements, closer to the foundation.
Technological Innovations in Earthquake Engineering
Seismic Monitoring and Sensors
Modern buildings are equipped with seismic monitoring systems that detect and analyze ground motion. These systems provide real-time data, enabling immediate action to protect occupants and the structure itself.
Smart Building Technologies
Advancements in technology have introduced smart materials and automated systems that adapt to seismic forces. For example, some buildings can adjust their damping mechanisms in real-time to counteract vibrations more effectively.
Lessons from Historical Earthquakes
Examining the performance of buildings during past earthquakes provides valuable insights into what works and what doesn't. Structures designed with modern engineering principles, such as Japan's high-rise buildings and California's retrofitted structures, demonstrate remarkable resilience.
Conclusion
The ability of some buildings to withstand earthquakes lies in a combination of innovative engineering, advanced materials, and thoughtful design. As we continue to learn from past disasters and refine our techniques, we can create safer structures that protect lives and property.
For more information, Please watch the short video tutorial